Features
Update 2021/7/30 The Health of Water
The molecule of life and aquaporin channels
Suzanne Forcese
All life, be it human, animal, botanical, microbial exists only by the elegant orchestration of Nature’s architecture – the organism’s ability to transport water.
To deal with constantly changing metabolic demands and surrounding conditions, cells need to exchange water rapidly between their cytoplasm (inside) and the external environment (outside).
For decades, science had speculated that microscopic pores might somehow facilitate movement of molecules -- like water --across biological membranes. However, it was not until 1992 that Peter Agre’s team, quite by chance, discovered the secret of the water channel.
In 2003, Peter Agre and his colleagues at the Johns Hopkins University, Baltimore, went on to receive the Nobel Prize in Chemistry for the discovery of the “first” water channel while working on red blood cell membrane proteins. Originally named CHIP28, this first channel is now known as Aquaporin 1.
While research continues on aquaporins, hundreds have now been identified in all life forms.
To learn about the unique symbiosis of water and the aquaporins, WATERTODAY spoke with Uwe Hacke, Professor of Functional Plant Biology, University of Alberta.
“Water is an essential component of almost all physiological and biochemical reactions; therefore, its presence is required everywhere within an organism,” Hacke tells us. “Water can diffuse through the lipid bilayer, but without aquaporins flow across membranes is slow.”
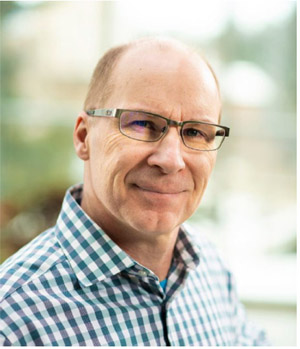
In the Hacke Lab, Dr. Uwe Hacke oversees research in plants and water.
“We also study how plants cope with stress factors such as climate change.”
Aquaporins (AQPs) are integral membrane proteins.
“The aquaporin family is ancient as evidenced by their presence in bacteria and fungi. Currently there are 13 identified aquaporins found in humans. Plants have 35 or more, all with specialized functions.”
Aquaporins are found in abundance in our human bodies in the membrane of red blood cells where they are used to control volume and pressure. They are also found in the cells of the kidney where they are used to quickly and efficiently reabsorb water from the filtrate (urine). When we produce tears, and saliva it is the aquaporins that help us release water as quickly as possible.
“They also facilitate skin hydration, and play a role in water balance of the central nervous system and in neuroexcitatory processes. In the eye, aquaporins are involved in lens transparency, intraocular pressure regulation and visual signal transduction,” Hacke adds.
“In addition, malfunction of mammalian aquaporins has been implicated in diverse deficiencies and diseases.”
Different cancers, edema, brain injury, obesity, glaucoma, Type 2 diabetes, skin diseases, sepsis, are among the pathologies that are included in aquaporin dysfunction and mutation.
Understanding the structure of aquaporins may lead to courses of action in limiting both non-life -threatening and life-threatening pathologies.
Interestingly, the structure of the aquaporin channel is the same in all organisms.
“The structure of the channel is important because it determines which molecules can pass, which are excluded and at which rate molecules can move through the pores.”
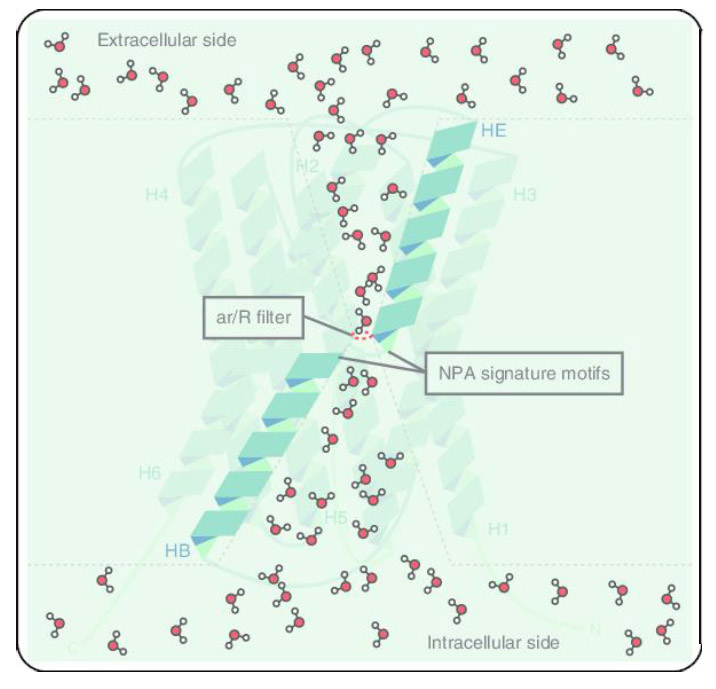
Aquaporins belong to the highly conserved major intrinsic protein (MIP) super family. MIPs exist as tetramers. Each subunit is behaving as a single aqueous pore. Sub units share the same hour-glass structure consisting of 6 transmembrane domains connected by several loops.
--Uwe G Hacke, Joan Laur, Aquaporins: Channels for the Molecule of Life
Hacke tells us that the key feature of water permeation is in the narrow centre of the pore which has a minimum diameter of 2.8 angstrom. (One angstrom is equal to one ten-billionth of a meter or 0.1 nanometers)
“Almost matching the diameter of a water molecule.”
This unique fit enables an extremely efficient passage. So efficient in fact that more than one billion water molecules per second pass through the channel in single file, “like a coordinated dance,” Hacke explains. “Exceptionally high flow rates are possible because of fine-tuned interactions between water molecules and the molecules forming the channel.”
This computer simulation demonstrates how every second, a billion water molecules (red & white spheres) pass through a channel formed in the middle of an aquaporin protein-- as shown by white (nonpolar) and green (polar) areas, and charged areas in blue (positive) and red (negative). The yellow sphere highlights the path of a single water molecule.
Water Channels in Cell Membranes
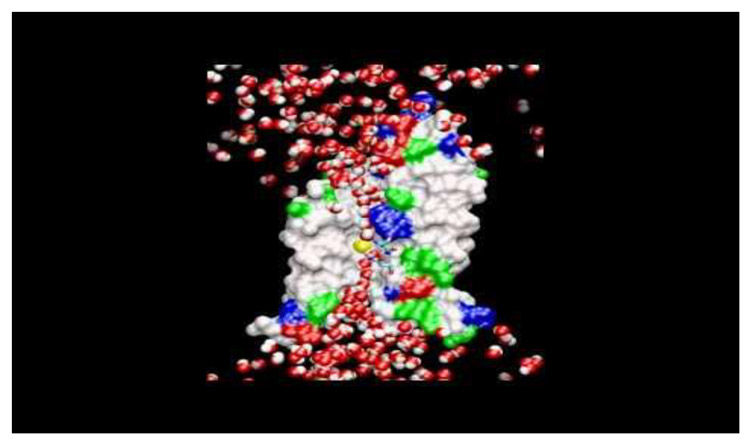
Can other molecules pass through the aquaporin channel?
“They would have to be small enough,” Hacke adds.
Some are physiologically important while others are toxic. In addition to the transport of water, aquaporins can facilitate the transcellular movement of glycerol, nitrate; urea and small neutral molecules such as CO2, ammonia, hydrogen peroxide; and metalloids such as boron, arsenic, antimony, silicon; and mercury.
Water permeation through aquaporins is a passive process that follows the direction of osmotic pressure across the membrane. Although many aquaporins function as always-open channels, a subgroup of aquaporins, particularly in plants have evolved a sophisticated molecular mechanism, called gating, through which the channel can be closed in response to harsh conditions of the environment such as drought stress and flooding which trigger certain cellular signals that result in closure of the channel.
“It has been observed in times of drought, for example, that a tree will know exactly how much water it will release through transpiration in the leaves and flowers, and how much to hold onto in the roots.”
In an interesting form of aquaporin regulation, researchers in the field of plant biotechnology have been able to modify the level of gene expression, by altering the number of proteins in an effort to produce drought resistance.
“With the fall-out from climate change this is certainly an interesting avenue of research,” Hacke says. “We already know the genes. We could tweak them to ensure our food security.”
The gating process has also inspired research into pharmaceuticals. For example, the kidneys are responsible for maintaining water balance in the body. Identifying a chemical that could close the aquaporins in the kidneys could be developed into a diuretic drug. Similarly, stabilizing the closed structure could be used in cancer treatment. Open aquaporins are necessary for cells to be able to move and form new blood vessels -- which tumors are dependent upon to grow. The discovery of a compound that would close the gateway would inhibit growth.
Many intriguing questions are still unresolved. It is such a tiny pore that has been with us since ancient times and yet we can learn so much by mimicking the intelligence of Nature.
suzanne.f@watertoday.ca
|